Particle size modification during drug development can significantly impact the dissolution and bioavailability of a drug. With increasing numbers of complex drug molecules in the development pipeline, presenting a hurdle for the pharmaceutical industry to overcome1,2. Particle size modification is therefore a crucial step for pharmaceutical companies to incorporate into their drug development process.
Milling, Micronization and Particle Modification of Pharmaceuticals
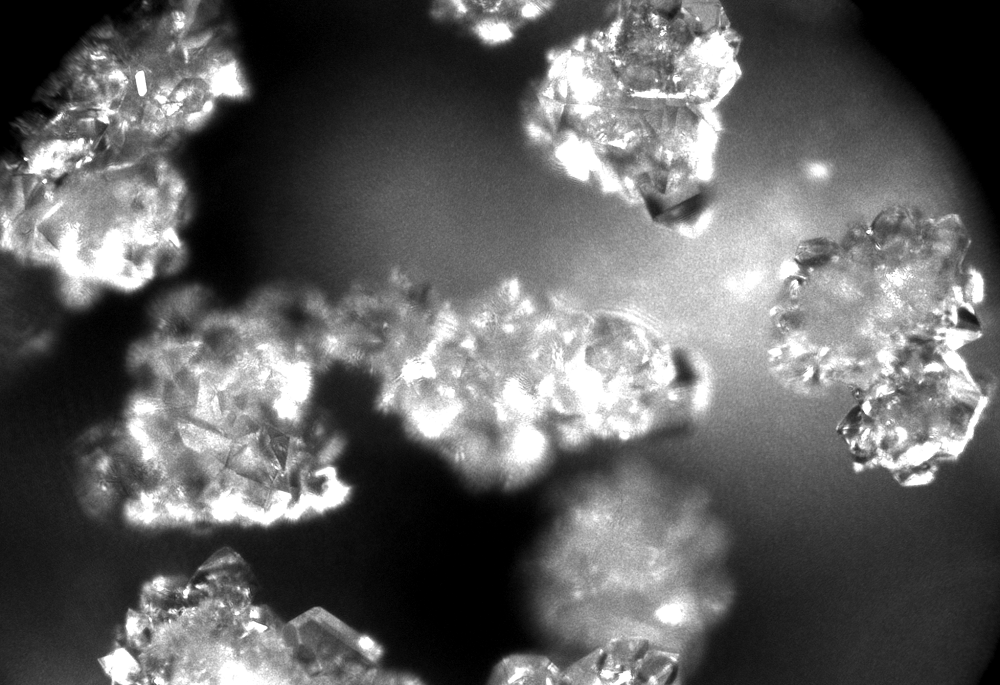
As well as improving solubility profiles, control over particle size is important in generating material with uniform particles. This facilitates dose consistency in the final drug product, which is especially important for highly potent APIs and/or low dosage formulations. Depending on the administration route and dosage form, there may also be a requirement for tight and specific particle size distributions, this is true for formulations for inhalation and ocular suspensions. Milling can also be used to improve the bulk properties of the powder such as flowability, bulk density and compressibility. The particle size and shape also affect processing of the API and can help or hinder filtrations, tabletting and excipient blending. Ensuring the efficient performance of an API from manufacturing to formulation is crucial in successfully bringing a drug to the market and once there, on its desired and consistent pharmacological performance upon administration in the body.
Particle size can be controlled by either a bottom-up approach, such as spray drying or crystallisation to give desired particle size / shape directly, or more commonly by top-down approaches such as milling. Milling can be split into two categories, wet and dry milling, each of which have distinct benefits and operating parameters. The correct milling technology is selected based on consideration of the solid-form, bulk properties, and desired particle size.
Dry Milling
Dry milling is the process of reducing particle size in the absence of a liquid milling media. Examples of types of dry milling are pin milling, hammer milling and jet milling. Pin milling causes particle breakage via impingement between stationary and rotating pins and can typically allow access to particles in the range of 10‑40 µm. Hammer milling causes breakage by impacts with rotating hammers accessing particles from 25-200 µm. Jet milling, often referred to as micronization, relies on high pressure gas within a milling chamber to facilitate breakage via particle-particle collision and collision of particles with the chamber. This can yield particles with sizes from 1-15 µm and as such has become an industry standard to obtain micronized material.
Figure 1: SEM images of APIs before and after micronization
Dry milling is particularly attractive as the lack of solvent alleviates concerns of solvent mediated solid-from conversion and residual solvent content. Solid form conversions/amorphization are sometimes observed due to the energies involved in particle breakage and therefore the underpinning solid form knowledge is essential to monitor and understand any transitions that may be observed. Jet milling in particular has been proven to be highly scalable with processes developed on the small scale easily transferred to kilo and production scale mills. Yields from micronization processes are very high, this is particularly important as it is typically the final step before formulation. Losses in yield are more costly the further into a multi-step synthesis/process and thus a high yield final step is key to ensure an efficient manufacturing process. The particle size distribution of the material obtained from jet milling can be fine-tuned by optimization of the feed rate, injection gas pressure and grinding gas pressure.
Wet Milling
Wet milling is characterized by suspension of the API in a liquid media. Wet milling is the best option for sensitive hydrates and APIs that would likely generate static charge build up during dry milling. Particle size is reduced by shearing, impact and crushing as the suspension passes through rotor-stators. Typically, wet milling in this manner can reduce particle size to between 10 and 100 μm depending on the material friability, the rotor-stator configuration and mill operating parameters.
Figure 2 IKA Ultra-Turrax used for wet milling parameter screening
An advantage of this type of milling is it can be set up as an inline process during a crystallization, allowing an additional control on the particle properties during the process. Alternatively, the suspension from a completed crystallization can be telescoped into a milling process before filtration, facilitating the formation of particles with both the desired size and morphology. Particle size distributions obtained from wet milling can be tuned toward a desired size by editing parameters such as rotor speed, rotor-stator gap and turnovers (passes through the mill).
A subset of wet milling is ball or bead milling where the suspension of API is added to a milling chamber with a milling media, typically very small ceramic balls (down to 0.1 mm bead diameter). Force is then imparted to the milling chamber, depending on the set up of the mill, which is transferred to the milling mixture causing particle breakage during collisions. Bead milling can generate sub-micron particles and nanosuspensions (200 to 300 nm). One additional advantage of ball/ bead milling is that it can be evaluated on relatively small scales (100’s mg) which makes it amenable to early R&D exploration. The effect of particle reduced API material can subsequently be evaluated in pharmacokinetic studies which can help direct target particle sizes for an API.
Figure 3: Ball mill mechanism of action (Khadka, et al., 2014)
Managing Solid Form and Particle Engineering with Confidence
The right choice for particle size modification is dependent on the unique properties of each API in development. With the success of the process dependent on a complex range of factors, expert support is crucial in identifying the right method.
With state-of-the-art equipment and a wealth of specialist knowledge, Veranova’s experts can provide customers with world-leading particle size modification spanning small R&D to manufacturing (cGMP) scales, backed by in-house solid form and analytical services to support drug candidates through the developmental pipeline.
References:
- Amidon, G. L., Lennernäs, H., Shah, V. P. & Crison, J. R., 1995. A Theoretical Basis for a Biopharmaceutic Drug Classification: The Correlation of in Vitro Drug Product Dissolution and in Vivo Bioavailability. Pharmaceutical Research, 12(3), pp. 413-420.
- Lipinski, C. A., 2000. Drug-like properties and the causes of poor solubility and poor permeability. Journal of Pharamacological and Toxicological Methods, 44(1), pp. 235-249.
- Khadka, P. et al., 2014. Pharmaceutical particle technologies: An approach to improve drug solubility, dissolution and bioavailability. Asian Journal of Pharmaceutical Sciences, 9(6), pp. 304-316.
About the author
Daniel Rixson (Ph.D., MChem.) is a scientist working in Veranova’s Solid Form and Particle Engineering centre of excellence based in Cambridge, UK. Dr. Rixson has extensive experience in crystallization development and particle engineering as well as being a keen crystallographer. Dr. Rixson received his doctorate from the university of Bath, UK in the field of metal-organic frameworks.